Way above my pay-grade…
To get my head around this – or try to – I wondered how many nanoparticles it would take to make a common garden variety pea… Here’s the maths:
We’ll need to make some assumptions about the size of a nanoparticle and the size of a pea. For simplicity’s sake, we’ll consider a nanoparticle as a sphere with a diameter of 1 nanometer (nm), or 1 billionth of a meter, and a pea as a sphere with a diameter of 1 centimeter (cm), or 1 hundredth of a meter.
- First, to calculate the volume of a pea and a nanoparticle.
The volume V of a sphere can be calculated using the formula V = 4/3 * π * r³, where r is the radius of the sphere.
For the nanoparticle: r_nano = 0.5 nm = 0.5 * 10^-9 m V_nano = 4/3 * π * (0.5 * 10^-9 m)³ ≈ 5.24 * 10^-28 m³
For the pea: r_pea = 0.5 cm = 0.5 * 10^-2 m V_pea = 4/3 * π * (0.5 * 10^-2 m)³ ≈ 5.24 * 10^-7 m³
- Then, divide the volume of the pea by the volume of a nanoparticle to find out how many nanoparticles would fit in the pea.
Number of nanoparticles = V_pea / V_nano ≈ 5.24 * 10^-7 m³ / 5.24 * 10^-28 m³ ≈ 10^21
So, it would take approximately 1 sextillion (10^21) nanoparticles to make a pea, according to these approximations and assumptions about size.
Are you any the wiser? 🙂
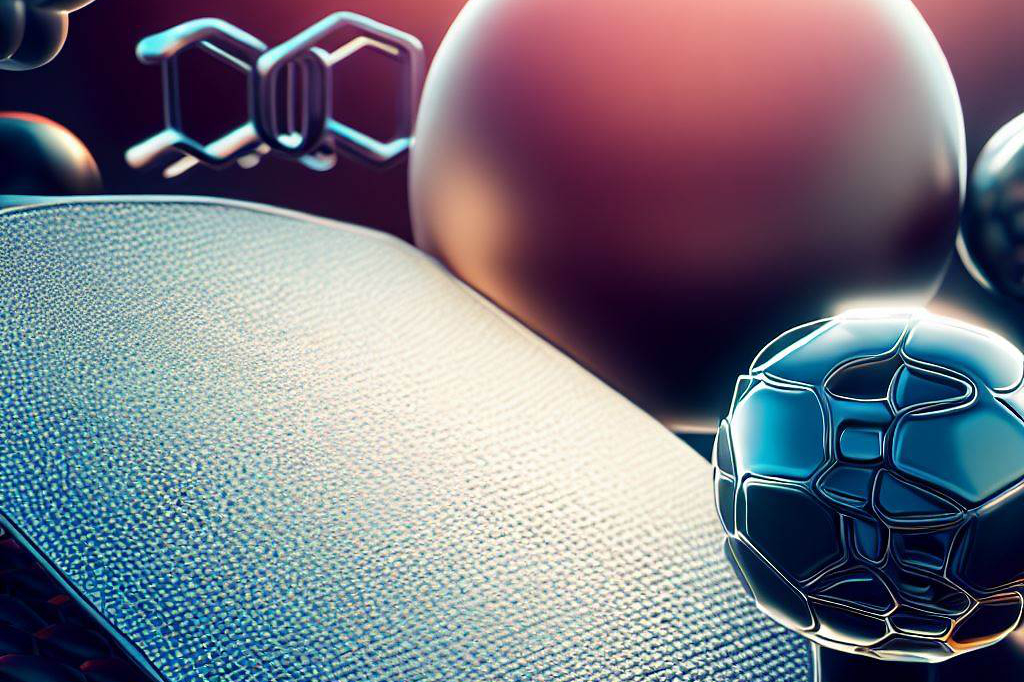
Nanotechnology is rapidly growing as a field of research and development, with numerous applications in various fields such as
- medicine,
- electronics,
- energy,
- and environmental science.
This progress is fueled by the ability to manipulate matter at the nanoscale level.
The word “nano” comes from a Greek word that means dwarf or little, so nanomaterials are materials that have at least one dimension in the range of 1-100 nanometers (nm).
Definition of Nanomaterials
Nanomaterials are materials with unique properties that emerge from their small size. As a result of their small dimensions, they often exhibit unusual and desirable physical, chemical, and biological properties that differ from larger scale counterparts.
For example, nanoparticles have a much greater surface area per unit mass than bulk materials; therefore, they can be highly reactive to their environment. Carbon-based nanomaterials like fullerenes and carbon nanotubes have high mechanical strength due to strong covalent bonds between carbon atoms.
Importance of Nanomaterials in Modern Science and Technology
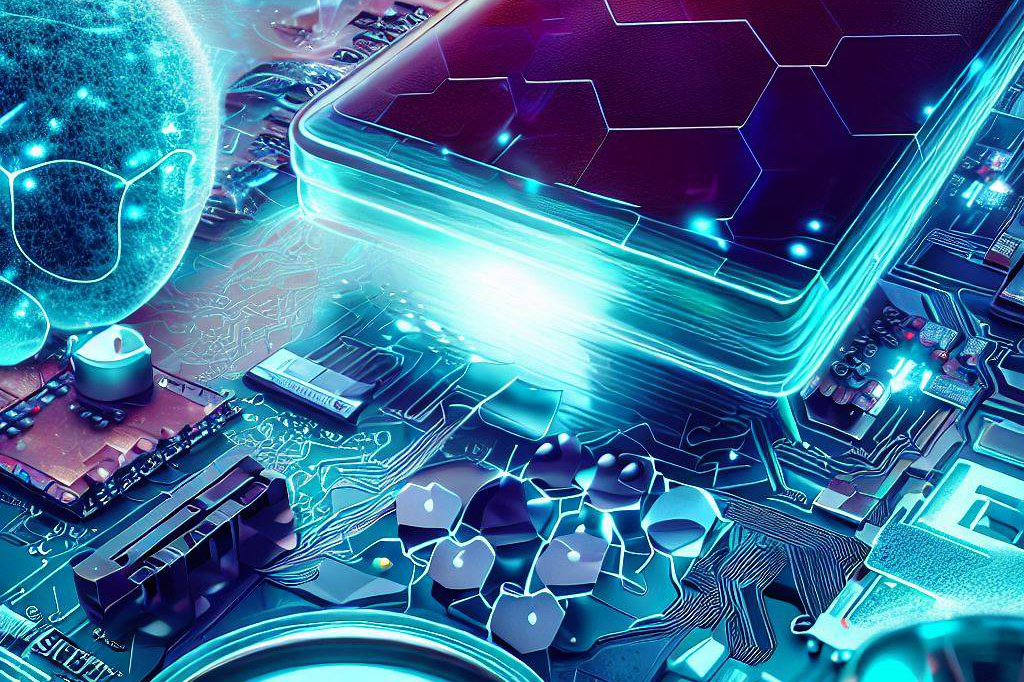
Nanotechnology has revolutionized modern science and technology by creating opportunities for developing innovative products that are
- smaller,
- faster,
- lighter
- or stronger
than traditional materials. The potential benefits are vast-ranging; more efficient solar cells for energy production, more effective drug delivery systems for medicine, or new electronic devices with superior performance can be developed using nanotechnology. The successful application of nanotechnology in various fields has increased demand for innovative breakthroughs in this area.
In fact, it’s expected that the global market for nanotechnology will reach over $125 billion by 2024. With such potential benefits on offer, there is no doubt why many governments and private entities are investing significant amounts of resources in nanotechnology research.
Overview of the Atomic Scale
The atomic scale is the scale at which matter is composed of atoms, which are the basic building blocks of everything around us.
The atom consists of a nucleus which contains protons and neutrons, surrounded by electrons that orbit the nucleus. At this atomic scale, quantum mechanics becomes important in understanding how these atoms behave.
It is at this level that we can begin to understand the fundamental structures of matter. Nanomaterials have unique properties due to their size and structure that make them highly desirable for various applications in science and technology.
The atomic-scale provides an essential understanding for manipulating materials at this level. In subsequent sections, we will delve into what makes nanoparticles so fascinating and explore some techniques used to study them.
The Fascinating World of Nanomaterials
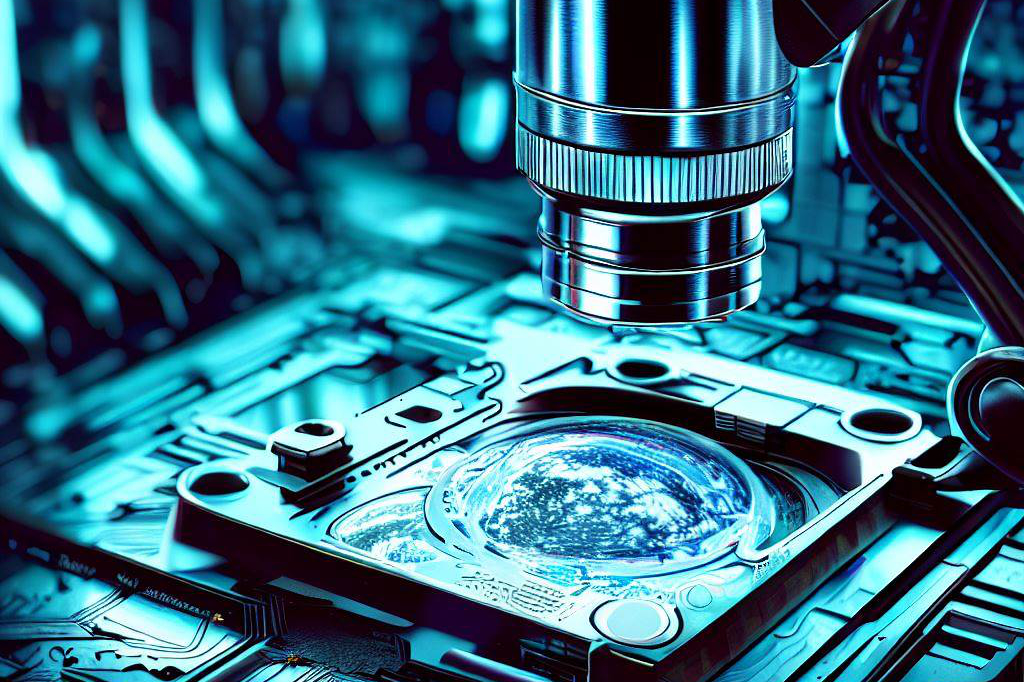
Properties and Characteristics of Nanomaterials
Nanomaterials possess unique physical, chemical, and mechanical attributes that are dissimilar from their bulk materials due to their small size and high surface-to-volume ratio. They exhibit remarkable
- optical,
- electrical,
- thermal,
- magnetic,
- and mechanical properties
that make them ideal for many applications in a broad array of fields.
For instance, nanoparticles have higher melting points than the corresponding bulk material; they can also act as catalysts for various chemical reactions. Also, the optical properties of nanomaterials can be fine-tuned by changing the particle size or shape.
Another fascinating property is their significantly high surface area which enables them to interact with other molecules or surfaces more dominantly than bulk materials. This characteristic makes nanomaterials suitable for drug delivery systems, as they can efficiently deliver therapeutic agents directly to targeted cells in the body.
Types of Nanomaterials (Carbon-based, Metal-based, Ceramic-based)
There are three categories of nanomaterials:
- carbon-based nanomaterials (such as carbon nanotubes and graphene),
- metal-based nanomaterials (such as silver nanoparticles),
- and ceramic-based nanomaterials (such as titanium dioxide).
Each type has unique characteristics that make them suitable for different applications.
Carbon-based nanomaterials have excellent electrical conductivity and mechanical strength, which makes them highly useful in applications like electronics and energy storage devices.
Metal-based nanoparticles are widely used in industries due to their antimicrobial properties; for example, silver nanoparticles are commonly used in textiles to produce self-cleaning fabrics.
Ceramic-based nanoparticles possess exceptional hardness, which makes them useful components in building materials like paints and coatings.
Applications and Uses of Nanomaterials (Electronics, Medicine, Energy)
The application areas for nanomaterials are vast and diverse, ranging from electronics to energy, medicine, and environmental remediation. In electronics, nanomaterials are used to produce high-performance transistors and sensors.
They hold great potential for future developments in nanoelectronics like quantum computing. In medicine, nanoparticles have been used in drug delivery systems due to their ability to target cancer cells precisely.
They can also be used in medical imaging techniques such as magnetic resonance imaging (MRI).
Nanotechnology is a game-changer in the field of renewable energy. For example, the use of nanocoatings on solar panels can increase their efficiency by reducing reflection losses.
These examples illustrate how nanotechnology is making a significant impact on various industries; it’s essential to continue exploring ways to leverage these materials’ unique properties and characteristics for future technological advancements.
Understanding the Atomic Scale
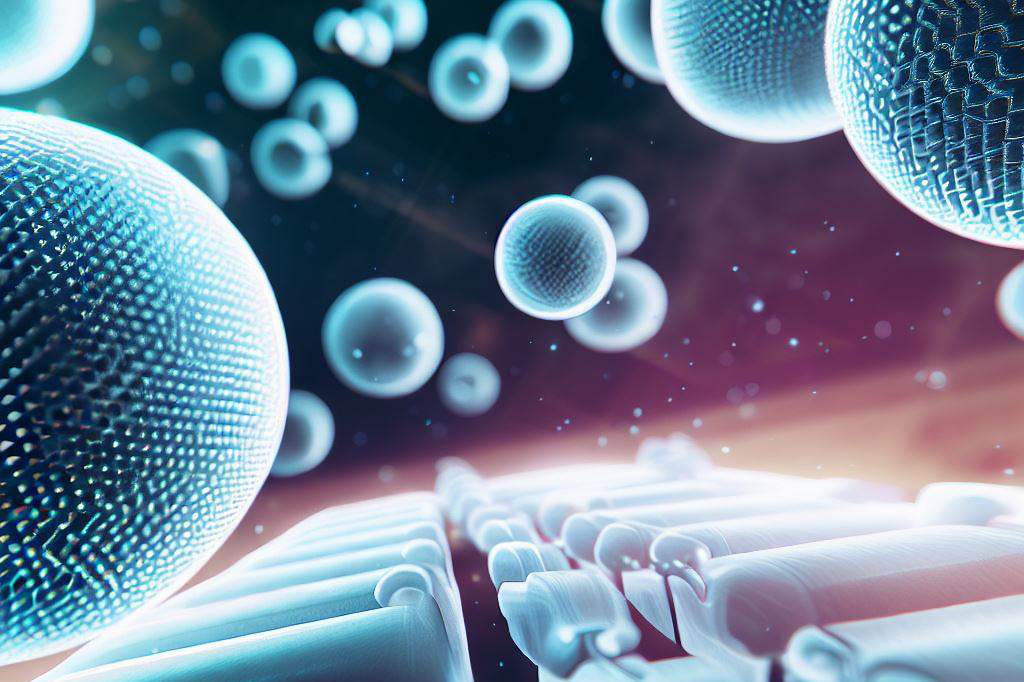
Fundamentals of Atomic Structure
Atoms are the basic building blocks of all matter, and they consist of three types of particles:
- protons,
- neutrons,
- and electrons.
Protons are positively charged particles found in the nucleus of an atom, while neutrons have no charge and also reside in the nucleus.
Electrons are negatively charged particles that orbit around the nucleus.
The number of protons in an atom’s nucleus is known as its atomic number, while the total number of protons and neutrons is its atomic mass.
Different elements have different numbers of protons in their nuclei, giving each element a unique atomic number. The periodic table arranges elements based on their atomic structure and chemical properties.
Quantum Mechanics and its Role in Understanding the Atomic Scale
Quantum mechanics is a branch of physics that studies matter at very small scales, such as atoms and subatomic particles. This field has been crucial to understanding atomic structure because it provides insights into how electrons behave within atoms. One important concept in quantum mechanics is wave-particle duality, which suggests that electrons can exhibit both particle-like and wave-like behaviors depending on how they are observed or measured.
Another concept is quantum superposition, which states that an electron can exist in multiple energy states simultaneously until it is observed or measured. These principles play a role in understanding the behavior of electrons within atoms and help scientists explain why certain elements have specific electronic configurations.
Techniques for Studying the Atomic Scale (Scanning Electron Microscopy, Transmission Electron Microscopy)
Scientists use various techniques to study atoms at extremely small scales.
One commonly used method is scanning electron microscopy (SEM), which involves firing a beam of electrons at a sample to create images with high resolution.
Transmission electron microscopy (TEM) is another method used to study atoms. This technique involves passing a beam of electrons through a thin sample, creating an image based on the electrons that pass through.
Other methods include X-ray crystallography, which involves firing X-rays at a crystal to determine the arrangement of atoms within it, and atomic force microscopy (AFM), which uses a small probe to scan surfaces at the atomic scale.
These techniques have allowed scientists to gain unprecedented insights into atomic structure and behavior.
Synthesis and Fabrication Techniques for Nanomaterials
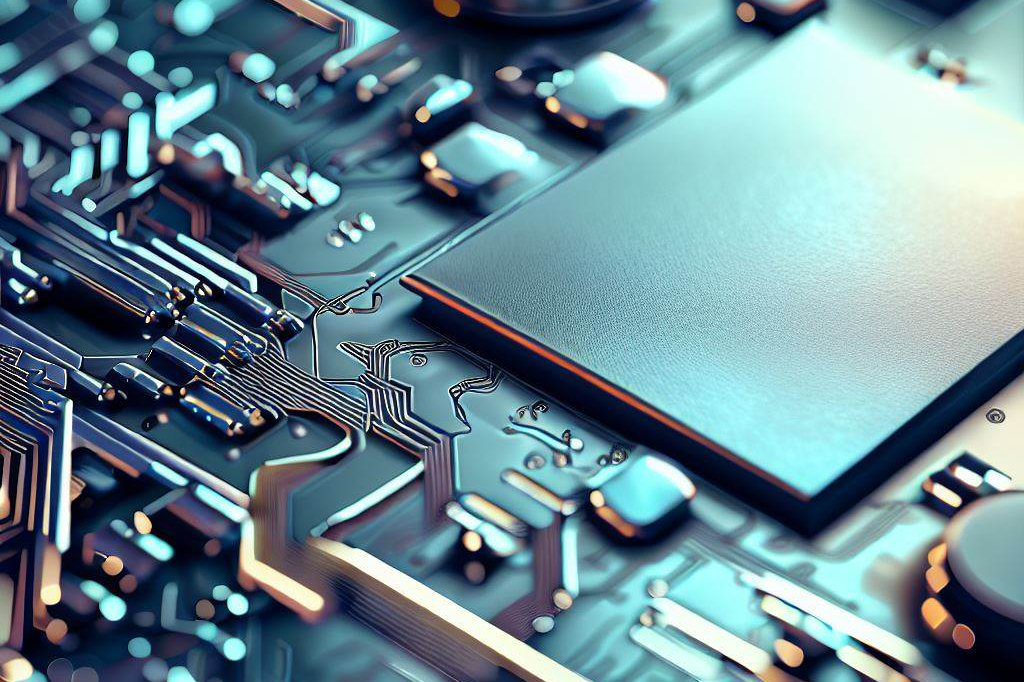
Top-Down Approach: Lithography and Etching
One of the most widely used techniques for synthesizing nanomaterials is known as the “top-down approach.” This approach involves breaking down larger materials into smaller ones, all the way down to the nanoscale. Two popular methods of doing this are lithography and etching. Lithography involves using a patterned mask to selectively remove material from a larger substrate, leaving behind a smaller structure.
This process is commonly used in semiconductor fabrication, where it allows for precise control over features on a microscopic scale.
Etching, on the other hand, involves using chemicals to selectively remove material from a larger substrate.
This can be done using wet or dry etching techniques, and can be used to create structures with very high aspect ratios (i.e., structures that are very tall and thin).
While lithography and etching are powerful tools for creating nanostructures with high precision, they do have some limitations.
For example, they are often limited in terms of the shapes that can be created (since they rely on masks or patterns). Additionally, they can be expensive and time-consuming processes.
Bottom-Up Approach: Chemical Vapor Deposition and Sol-Gel Synthesis
Another common approach to nanomaterial synthesis is known as the “bottom-up approach.” This approach involves building up small structures into larger ones until you reach the desired size at the nanoscale. Two popular methods of doing this are chemical vapor deposition (CVD) and sol-gel synthesis.
CVD involves introducing gases into a reaction chamber where they react with a substrate to form solid materials.
By carefully controlling factors such as temperature and pressure, it’s possible to create very small particles with precise shapes and sizes.
Sol-gel synthesis, on the other hand, involves using a liquid precursor that undergoes a chemical reaction to form a solid material. This process is often used to create materials like ceramics and glasses at the nanoscale.
One advantage of the bottom-up approach is that it allows for more flexibility in terms of the shapes and sizes of structures that can be created.
Additionally, it can be less expensive than the top-down approach (particularly for large-scale production). However, it can also be more difficult to control the synthesis process and ensure reproducibility.
Overall, both top-down and bottom-up approaches have their advantages and disadvantages when it comes to synthesizing nanomaterials. The choice of which technique to use will depend on factors such as the specific application, desired properties of the final material, and available resources.
Challenges and Risks Associated with Nanomaterials
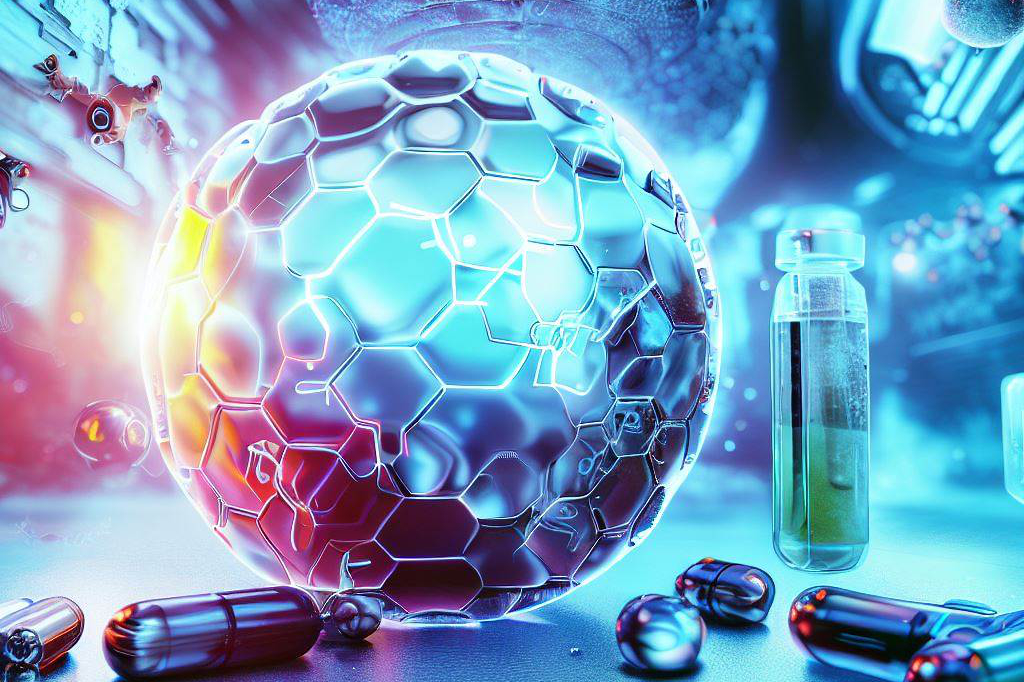
Environmental Impacts
Nanomaterials have unique properties and characteristics that contribute to their widespread use in various applications. However, these same properties can also lead to environmental impacts when nanomaterials are released into the environment. One of the significant risks associated with nanomaterials is their potential toxicity to living organisms.
Due to their small size, they can enter into and accumulate in the tissues and organs of organisms, causing damage. Furthermore, nanomaterials have been found to be persistent in the environment, meaning they do not break down quickly like other materials.
These materials can accumulate in soil or water systems, leading to contamination of food sources or drinking water.
The long-term effects of exposure to nanomaterials on ecosystems are still being studied.
To address these concerns, researchers are exploring how nanomaterials move through different environmental compartments and how they interact with other substances present in the environment. Developing appropriate regulations and guidelines for the use of nanomaterials is also necessary to minimize potential environmental impacts.
Toxicity Concerns
While there are many benefits associated with the use of nanomaterials, there is also a concern about their toxicity due to their small size and unique physicochemical properties.
Traditional toxicology tests may not be effective when dealing with these materials because they might not identify specific hazards that arise at the nano-scale level. Scientists are still working on gaining a better understanding of how different types of nanoparticles interact with living tissues at various levels (cellular, organ-level) as well as determining whether or not these interactions lead to adverse health outcomes like inflammation or cancer development.
Regulators worldwide require extensive testing for any new drugs or compounds before approving them for human consumption; this testing process could take years before approval goes through government organizations such as FDA.
The same level of testing and precautionary measures must be in place for nanomaterials.
The Need for Regulation
There is an urgent need for the development of effective regulatory policies to ensure that the benefits of nanotechnology are not outweighed by its potential risks. Governments worldwide need to establish a prerequisite pre-market safety evaluation before any product containing nanomaterials is allowed to enter the market. This will ensure that public health and environmental safety concerns are adequately addressed.
Education on the potential risks associated with nanomaterials is also crucial to raise awareness among producers, consumers, and policymakers. This education will help identify hazards, provide recommendations on how to mitigate risks effectively, and ensure safe use across all sectors.
Although there are significant challenges and risks associated with the use of nanomaterials in various applications, their unique properties offer numerous possibilities for the advancement of science and technology. By appropriately addressing these challenges through regulation and research, we can reap the benefits while minimizing potential harms.
Future Prospects for Nanotechnology Research
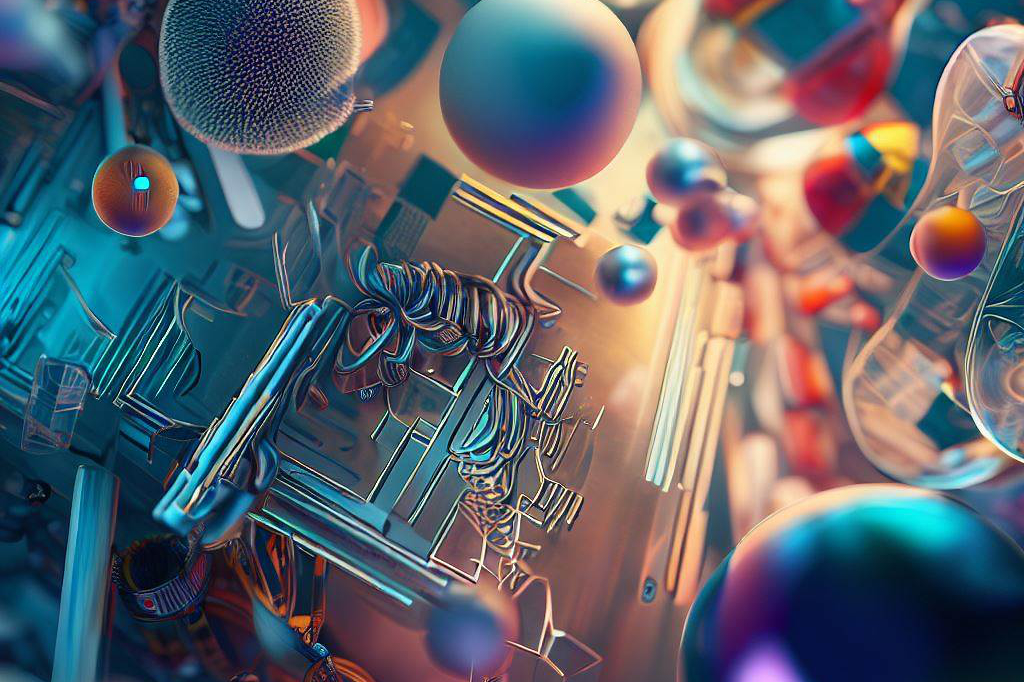
Revolutionizing Medicine with Nanomaterials
The future of nanotechnology research is bright, as it has the potential to revolutionize several fields, including medicine. Nanoparticles can be engineered to target specific cells or organs within the human body, paving the way for personalized medicine that is tailored to each patient’s unique needs.
For example, researchers are investigating the use of nanoscale drug delivery systems that can transport drugs and therapeutic agents directly to cancer cells while leaving healthy cells untouched.
This approach could minimize the side effects associated with traditional chemotherapy and significantly improve treatment outcomes. In addition, nanomaterials also hold great promise in the field of regenerative medicine.
Scientists are exploring the use of scaffolds made from biocompatible nanoparticles to support tissue growth and regeneration. This technology could enable patients suffering from injuries or degenerative diseases to regenerate damaged tissues and organs, potentially eliminating the need for surgery or organ transplants.
Nanoelectronics: The Future is Tiny
Another promising area of research is nanoelectronics – a field that involves designing electronic devices on an extremely small scale using nanometer-sized components. Miniature transistors and other electronic components that are only a few atoms thick have already been developed and are being integrated into everyday electronic devices such as smartphones and computers.
The potential applications of nanoelectronics are vast – from ultra-high-speed computing to extremely sensitive sensors capable of detecting tiny concentrations of chemicals in air or water.
As researchers continue to develop new materials and fabrication techniques for creating even smaller components, the possibilities for this emerging technology become increasingly exciting.
Nanotechnology as a Key Enabler for Sustainable Development
The Role of Nanomaterials in Renewable Energy
Nanomaterials have tremendous potential to contribute to sustainable development, particularly in the area of renewable energy. For example, scientists are exploring the use of nanoparticles to improve the efficiency of solar cells by enhancing light absorption and reducing energy loss due to heat.
This technology could significantly increase the amount of electricity generated by solar panels while reducing their cost. In addition, nanomaterials are being investigated for use in energy storage systems such as batteries and supercapacitors.
By utilizing nanoscale materials with high surface areas, researchers can create more efficient and compact devices that store more energy than traditional batteries. These advancements could pave the way for a future where renewable energy sources provide a larger portion of our global energy needs.
Nanotechnology and Sustainable Water Management
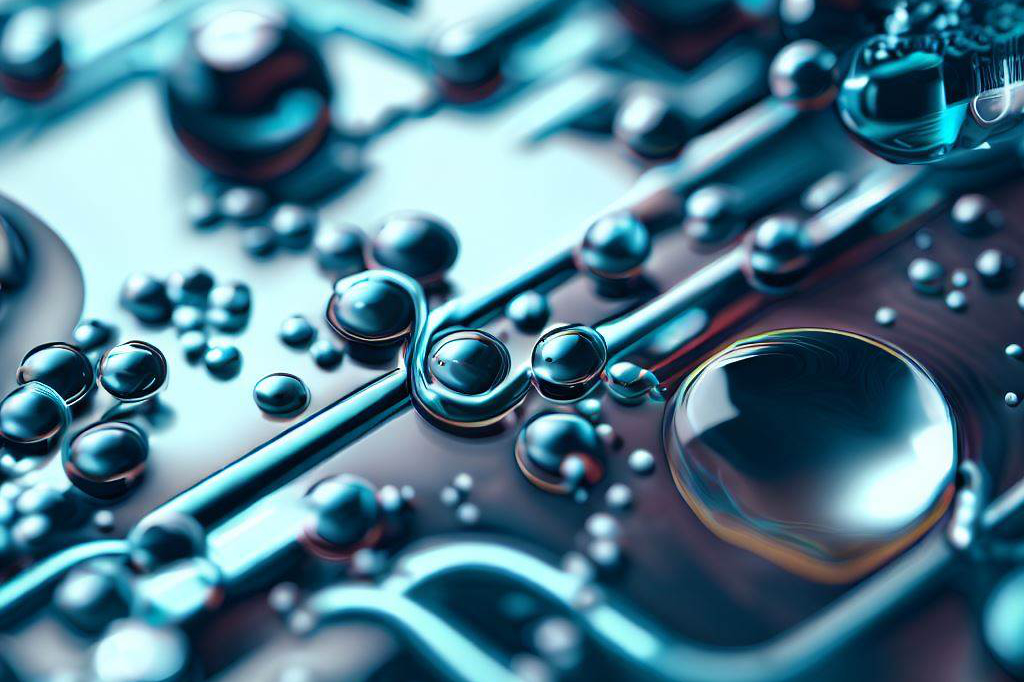
Another area where nanotechnology can contribute to sustainable development is water management. Nanoparticles can be used to remove contaminants from water and create highly effective filters that are capable of removing even tiny particles or impurities from water supplies. Moreover, scientists are researching ways to use nanoparticles to desalinate seawater and make it potable for human consumption.
This technology could help alleviate water scarcity issues in regions where freshwater is scarce but seawater is abundant. In addition, nanomaterials could also be used to monitor water quality in real-time, enabling faster response times in cases of contamination events or other emergencies.
Nanotechnology research holds tremendous promise for addressing some of the world’s most pressing challenges related to healthcare, renewable energy, and sustainable development. As this field continues to evolve and develop new materials and technologies, we can expect remarkable breakthroughs that have the potential to transform our world for the better.
Final Thoughts
Looking Ahead: The Future of Nanomaterials
Nanotechnology has been a rapidly growing field over the past few decades, with countless innovations and breakthroughs. The use of nanomaterials in medicine, energy, and electronics has revolutionized these industries. Researchers around the world are continuing to develop new techniques to produce and control nanomaterials, leading us into an era of unprecedented progress.
Looking ahead, it is clear that nanotechnology will continue to be a key enabler for sustainable development. The use of nanomaterials in green technologies such as solar cells and batteries can help reduce greenhouse gas emissions.
In medicine, targeted drug delivery using nanocarriers can optimize treatment efficacy while minimizing side-effects. These are just a few examples of the potential applications of nanomaterials in creating a more sustainable future.
The Importance of Responsible Nanotechnology
While the potential benefits of nanotechnology are immense, we must also acknowledge the challenges and risks that come with this field. As we continue to develop new materials with unprecedented properties, it is crucial that we prioritize safety and environmental protection.
This requires responsible research practices, transparent communication about potential risks associated with various materials or processes used in producing these nanomaterials. It is equally important for policymakers to establish regulations surrounding the production and use of these materials.
Nanomaterials have indeed unveiled wonders at atomic scale, which have revolutionized science technology across several industries. From material science to biomedical engineering to energy storage systems, the manufacturing process has become more efficient than ever before by utilizing these materials’ unique properties.
The future looks bright for those working on nanotechnology research and how they can further optimize its benefits while minimizing any adverse impact from their ecological footprint or toxicity concerns associated with them.
FAQ: Guide to Nanomaterials
1. What are nanomaterials?
– Nanomaterials refer to materials that have at least one dimension in the nanometer range, typically between 1 and 100 nanometers. These materials possess unique properties and characteristics due to their small size, which can differ from those exhibited by the same material at a larger scale.
2. What are the applications of nanomaterials?
– Nanomaterials find applications in various fields, including electronics, medicine, energy, and environmental science. They are used in the development of faster and smaller electronic devices, targeted drug delivery systems, efficient energy storage devices, and pollution remediation, among other applications.
3. How are nanomaterials synthesized?
– Nanomaterials can be synthesized through several methods, including bottom-up and top-down approaches. Bottom-up synthesis involves building the material atom by atom or molecule by molecule, such as in chemical vapor deposition or sol-gel techniques. Top-down synthesis, on the other hand, involves reducing the size of bulk materials through processes like grinding or etching.
4. What are the potential risks associated with nanomaterials?
– While nanomaterials offer numerous benefits, it is important to consider their potential risks. Due to their small size, nanomaterials may have different biological, chemical, and physical properties compared to their larger counterparts. It is crucial to conduct thorough research and risk assessments to ensure the safe use and disposal of nanomaterials and minimize any potential environmental or health hazards.
5. Are there regulations in place for the use of nanomaterials?
– Many countries have implemented regulations and guidelines regarding the use of nanomaterials to ensure their safe handling and minimize any potential risks. These regulations often focus on aspects such as labeling requirements, occupational safety measures, and environmental impact assessments. It is essential for manufacturers, researchers, and users to stay updated on the specific regulations applicable in their respective regions.
6. What are the advantages of using nanomaterials in electronics?
– Nanomaterials offer several advantages in the field of electronics. Their small size allows for the development of smaller and more efficient electronic devices with enhanced performance. Nanomaterials can improve the conductivity of materials, provide better thermal management, and enable novel applications such as flexible and transparent electronics.
7. Can nanomaterials be used in healthcare and medicine?
– Yes, nanomaterials hold great promise in healthcare and medicine. They can be used in targeted drug delivery systems, where drugs are encapsulated within nanoscale carriers to improve their efficacy and minimize side effects. Nanomaterials can also be utilized in medical imaging, tissue engineering, and diagnostics, enabling advancements in personalized medicine and improved patient care.
8. How do nanomaterials contribute to energy applications?
– Nanomaterials have significant implications for energy-related applications. They can be used in energy storage devices, such as batteries and supercapacitors, to enhance their capacity, charge/discharge rates, and overall performance. Nanomaterials are also employed in solar cells to improve light absorption and electron transport, leading to more efficient conversion of sunlight into electricity.
9. Can nanomaterials help address environmental challenges?
– Yes, nanomaterials have the potential to address various environmental challenges. They can be used in pollution remediation processes to remove contaminants from air, water, and soil. Nanomaterials with photocatalytic properties can break down pollutants under light irradiation. Additionally, they can enable the development of sensors for detecting environmental pollutants at low concentrations.
10. Are there any ethical concerns associated with nanomaterials?
– Ethical concerns surrounding nanomaterials include issues such as their potential impact on human health, the environment, and privacy. It is important to conduct thorough research and risk assessments to ensure the responsible development, use, and disposal of nanomaterials. Ethical considerations also include equitable distribution of the benefits and potential risks associated with nanotechnology.
TL;DR…
– 💡 Nanomaterials are defined as materials with at least one dimension in the nanometer range, possessing unique properties.
– 🔬 Nanomaterials play a crucial role in modern science and technology, finding applications in electronics, medicine, and energy sectors.
– 🌍 Understanding the atomic scale is essential for comprehending the fascinating world of nanomaterials.
– 🏭 Nanomaterials can be classified into different types such as carbon-based, metal-based, and ceramic-based materials.
– 💼 Nanomaterials have diverse applications in various fields, including electronics, medicine, and energy.
– 🔍 Studying the atomic scale requires techniques like scanning electron microscopy and transmission electron microscopy.
– 🧪 Nanomaterials can be synthesized using top-down approaches like lithography and etching, or bottom-up approaches like chemical vapor deposition and sol-gel synthesis.
– ⚠️ Nanomaterials pose challenges and risks, including environmental impacts, toxicity concerns, and the need for regulations.
– 🔮 The future of nanotechnology holds prospects for revolutionizing medicine, nanoelectronics, sustainable development, renewable energy, and water management.
– 👓 Responsible nanotechnology practices are crucial for ensuring the safe and ethical advancement of nanomaterials.
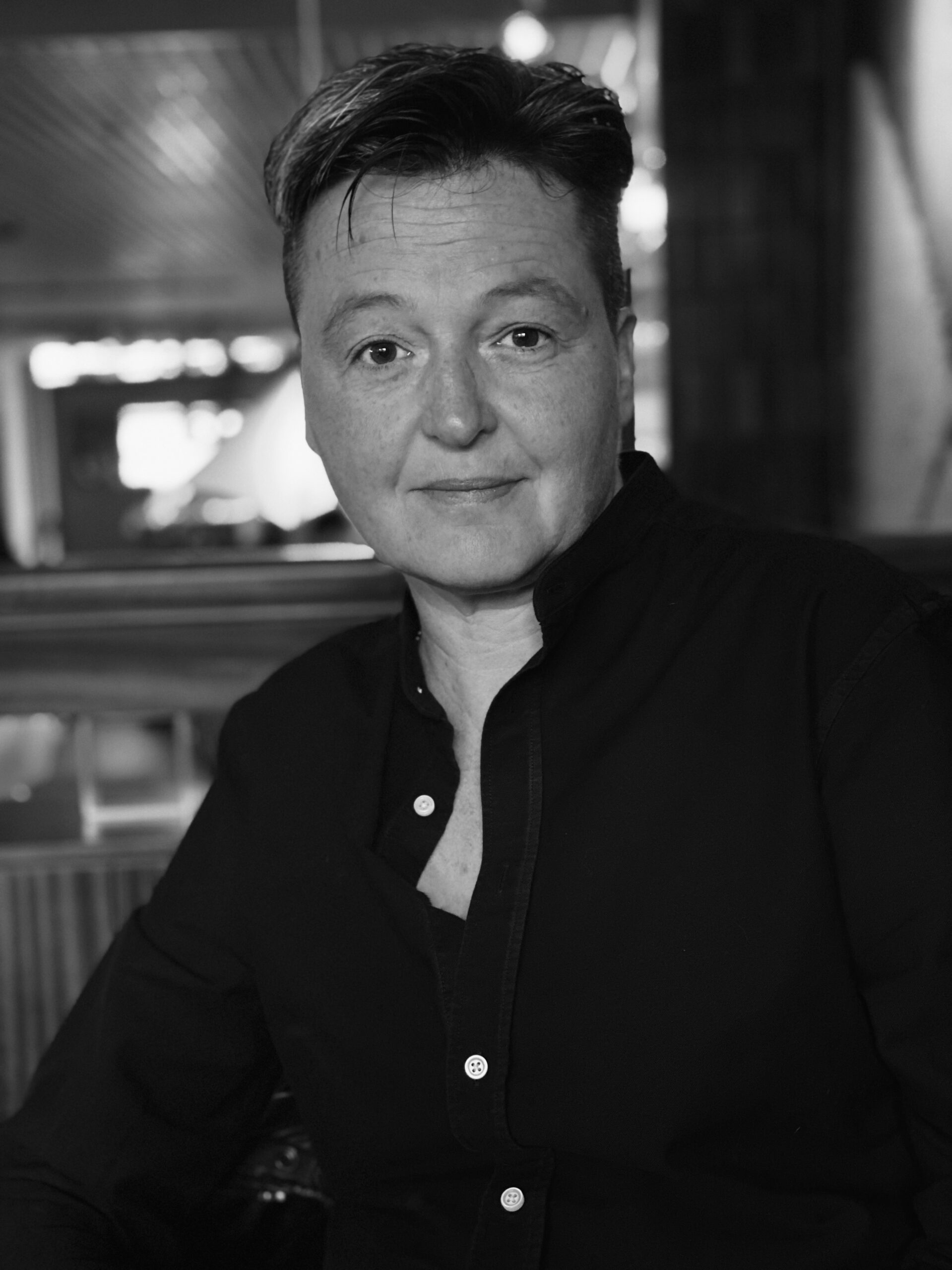
C M, a seasoned editor, journalist, and consultant, is deeply fascinated by the convergence of technology, space, and the future of humanity.
With a particular interest in transhumanism, futurology, and the philosophical and ethical dimensions of these domains, C M serves as the lead contributor to TranscendSphere and SpaceSpotlight.
When not penning insightful articles on these rapidly evolving fields, C M indulges in their love for podcasts and books, proudly embracing their status as a ‘Happy Nerd Extraordinaire!’